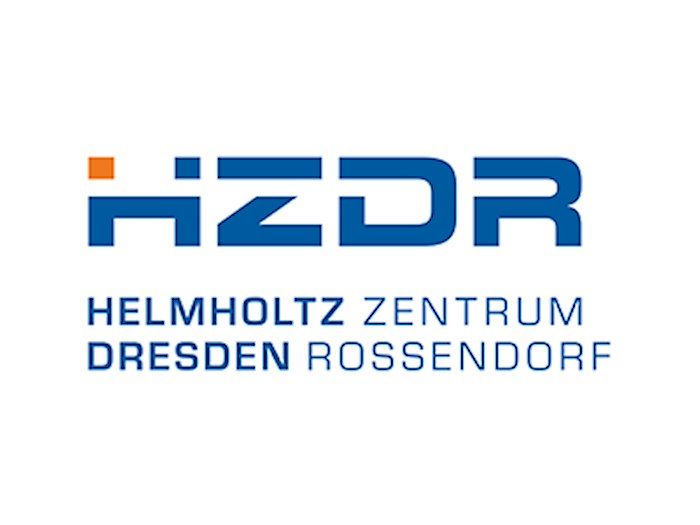
“Uranditelluride, or UTe2 for short, is a high-flyer among superconducting materials,” says Dr. Toni Helm from the High Field Magnetic Laboratory (HLD) at the HZDR. “It has been known since 2019 that the compound conducts electricity without loss – but in a different way to conventional superconductors.” Since then, research groups around the world have been interested in this material. This includes Helm’s team, which has now come a lot closer to understanding this material.
“To understand the hype surrounding the material, we need to take a closer look at superconductivity. This phenomenon is a result of the movement of electrons in the material,” explains the physicist, adding: “Because whenever they collide with the crystal lattice, they release energy in the form of heat. This is noticeable as electrical resistance. Electrons can avoid this by coming together in what are known as Cooper pairs.” This involves two electrons working together at extremely low temperatures to move through a solid without any friction. They can use vibrations of the atoms in their immediate vicinity as a kind of wave on which they can surf without losing energy. These atomic vibrations explain conventional superconductivity.
“For some years now, however, superconductors have also been known in which Cooper pairs form due to effects that are not yet fully understood,” says Helm. One possible form of unconventional superconductivity is spin-triplet superconductivity, which makes use of magnetic fluctuations. “There are also materials in which the outer electrons of the atoms come together collectively,” explains the physicist. “In this case, they not only shield the magnetism of the material, they also have an extremely high mass – for electrons.” These materials are known as heavy fermion superconductors. UTe2 could therefore be both a spin triplet and a heavy-fermion superconductor, as current experiments suggest. And it is also the heavyweight champion of the world. At the moment, no other heavy fermion superconductor is known that is still superconducting at higher magnetic fields. The experiments have also shown this.
Extremely robust in magnetic fields
Superconductivity is determined by two factors: the transition temperature and the critical magnetic field. If the temperature falls below the transition temperature, the resistance drops to zero and the material becomes superconducting. External magnetic fields also influence superconductivity. If these exceed a critical value, the effect collapses. “Physicists have a rule of thumb for this,” reports Helm: “In many conventional superconductors, the value of the transition temperature in Kelvin is roughly one to two times the value of the critical magnetic field strength in Tesla. In spin-triplet superconductors, this ratio is often much higher.” With their measurements on the heavyweight UTe2, the researchers have now been able to raise the bar even higher: At a transition temperature of 1.6 Kelvin (-271.55°C), the critical magnetic field strength is around 45 times higher at 73 Tesla – a record.
“Until now, heavy-fermion superconductors were of little interest for technical applications,” explains the physicist. “They have a very low transition temperature and the effort required to cool them is correspondingly high.” Their insensitivity to external magnetic fields could compensate for this shortcoming. This is because lossless current transport is mainly used today in superconducting magnets, for example in MRI scanners. However, the magnetic fields also influence the superconductor itself. A material that can withstand very high magnetic fields and still conduct electric current without loss would represent a major step forward.
Special treatment for demanding material
“Of course, UTe2 cannot be used to make a coil for a magnet,” says Helm. “Firstly, the material’s properties make it unsuitable for this, and secondly, it is radioactive. But it is perfectly suited to exploring the physics behind spin-triplet superconductivity.” Based on their experiments, the researchers developed a model that could serve as an explanation for superconductivity with extremely high stability against magnetic fields. To do this, they worked with samples just a few micrometres thick, which measure only a fraction of the thickness of a human hair (around 70 micrometres). The radioactive radiation emitted by the sample therefore remains much lower than that of the natural background.
In order to obtain such a tiny sample, Helm used a high-precision ion beam with a diameter of just a few nanometers as a tool. He used this to cut out the desired sample shape. As UTe2 is an air-sensitive material, Helm prepares his samples in a vacuum and then seals them with epoxy glue. “For the final proof that our material is a spin triplet superconductor, we would have to examine it spectroscopically while it is exposed to the magnetic field. However, current spectroscopy methods still fail at magnetic fields above 40 Tesla. Alongside other teams, we are also working on developing new ones. This would allow us to provide definitive proof,” says Helm confidently.
Publication
T. Helm, M. Kimata, K. Sudo, A. Miyata, J. Stirnat, T. Förster, J. Hornung, M. König, I. Sheikin, A. Pourret, G. Lapertot, D. Aoki, G. Knebel, J. Wosnitza, J.-P. Brison, “Field-induced compensation of magnetic exchange as the possible origin of reentrant superconductivity in UTe2” in Nature Communications, 2024 (DOI: 10.1038/s41467-023-44183-1)
Contact
Dr. Toni Helm
High Field Magnetic Laboratory Dresden at the HZDR
Tel: +49 351 260 3314
Email: t.helm@hzdr.de
– – – – – –
Further links
👉 www.hzdr.de
Photo: B. Schröder/ HZDR