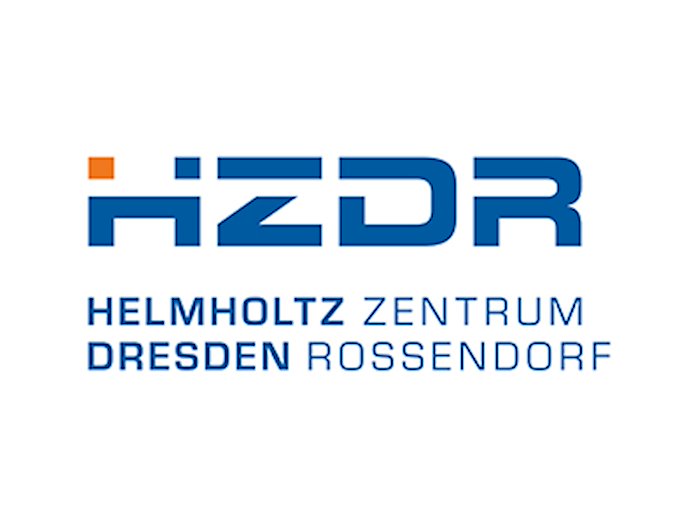
“An antiskyrmion is, in a sense, the antiparticle of the skyrmion. Both are so-called quasiparticles that owe their properties to the collective interaction of a large number of particles in the solid state and differ greatly from the properties of the underlying elementary particles,” says Dr. Toni Helm of the High Field Magnet Laboratory Dresden (HLD) at HZDR. Helm illustrates the coexistence of the two quasiparticles in a picture: “The microscopic skyrmion vortices form in a sea of magnetic particles within special materials and behave strangely. If a ‘magnetic’ navigator were to approach them, he would either be attracted or repelled. Antiskyrmions, on the other hand, would be nearly impossible for him to detect because these peculiar ‘anti’-vortices combine the different behavior of skyrmions.”
Characteristic footprint hidden in electrical signals
This analogy suggests that detecting antiskyrmions is quite difficult. But Helm’s team has followed a theoretical prediction that suggests a way to do so. Because of unique geometric properties – their so-called topology –Â antiskyrmions cause an additional voltage in the material’s electrical conduction. The team has now for the first time used electrical measurement methods in combination with magneto-optical microscopy to reveal the electrical signature of the antiskyrmions in the studied material.
The characteristic footprint of the magnetic antiskyrmions is hidden in the so-called Hall effect. In this effect, the current is deflected due to an external magnetic field applied perpendicular to the flow direction. Possible existing topological vortices generate a local magnetic field, which leads to an additional voltage. According to theory, this signal is directly interwoven with the respective topology of the vortices. Thus, by measuring the Hall effect, one could distinguish skyrmions from antiskyrmions. “Our study suggests that this contribution is extremely small and the measured signature is mainly due to the magnetic properties of the antiskyrmions. With our results, we help to better distinguish the actual Hall signature from other effects and give a first estimate of its magnitude, which refutes previous research results,” Helm concludes.
Scalable: the smaller, the more structured
Helm’s team targeted a particular magnetic compound of the metals platinum, manganese and tin, which belongs to the class of Heusler compounds, for the studies. These crystalline compounds exhibit different behavior than one would expect from their composition. For example, they are ferromagnetic, although none of their elementary building blocks is that in itself.
Under certain conditions, various topological structures such as skyrmions or antiskyrmions can form in the compound studied. And the scientists* noticed another fascinating detail: The strength of the expression of the antiskyrmions depends on and can be controlled by the sample thickness. “They are not detectable in a more massive piece of the starting material, but appear when the material is cut into flat platelets with thicknesses below ten micrometers,” Helm explains. To do this, the physicists use a type of ion beam gun with which they cut the crystals of the starting material into fine pieces.
For technological applications, scalability plays a crucial role. Nanoscale devices are needed, for example, to arrive at novel magnetic storage and data transmission systems based on such quasiparticles. Further properties of the material, which were researched in collaboration with colleagues from the HZDR Ion Beam Center, were incorporated into the supplementary theoretical calculations and simulations. With them, Helm’s team was able to substantiate the existence of antiskyrmions and show how precisely they can form from a highly complex magnetic environment.
Outlook
Helm sees potential for future applications in information technology: “Skyrmions are now being traded as candidates for quantum bits, or qubits for short, to handle data storage in topological quantum computers. If this works, it would apply equally to our antiskyrmions.”
From a technological point of view, materials in which these effects can be produced at lower magnetic field energies will become more important. Here, the Heusler compounds in particular are of interest, since they can be modified in an extremely wide variety of ways using targeted chemical processes.
Publication
M. Winter, F. J. T. Goncalves, I. Soldatov, Y. He, B. E. Zúñiga Céspedes, P. Milde, K. Lenz, S. Hamann, M. Uhlarz, P.Vir, M. König, P. J. W. Moll, R. Schlitz, S. T. B. Goennenwein, L. M. Eng, R. Schäfer, J. Wosnitza, C. Felser, J. Gayles, T. Helm, Antiskyrmions and their electrical footprint in crystalline mesoscale structures of Mn1.4PtSn, Communications Materials, 2022 (DOI: 10.1038/s43246-022-00323-6)
Contact
Dr. Toni Helm
High Field Magnet Laboratory Dresden at HZDR
Tel.: +49 351 260 3314
Email: t.helm@hzdr.de
About the Helmholtz-Zentrum Dresden-Rossendorf (HZDR)
The Helmholtz-Zentrum Dresden-Rossendorf (HZDR) conducts research in the fields of energy, health and matter. The following questions are in focus here:
- How to use energy and resources efficiently, safely and sustainably?
- How to better visualize, characterize and effectively treat cancer?
- How do matter and materials behave under the influence of high fields and in the smallest dimensions?
The HZDR develops and operates large infrastructures that are also used by external measurement guests: Ion Beam Center, High Field Magnetic Laboratory Dresden and ELBE Center for High Power Radiation Sources. It is a member of the Helmholtz Association, has six sites (Dresden, Freiberg, Görlitz, Grenoble, Leipzig, Schenefeld near Hamburg) and employs nearly 1,500 staff* – of which about 670 are scientists* including 220 PhD students.
– – – –
Further links
👉 www.hzdr.de
Photograph: B. Schröder/HZDR