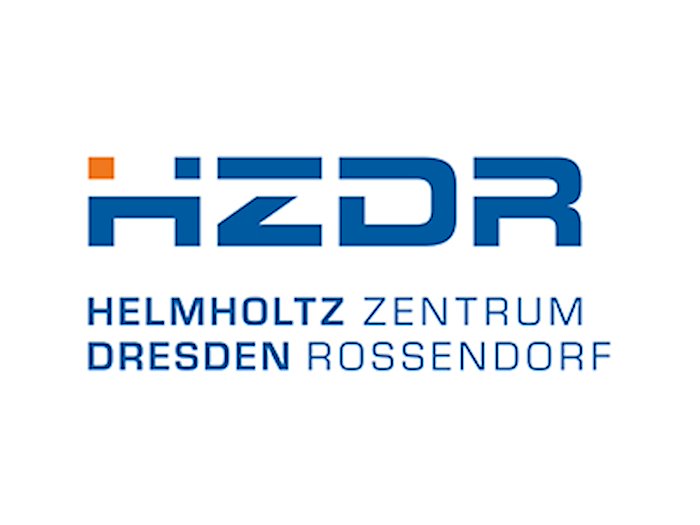
The computer codes used by researchers around the world form the basis for tens of thousands of scientific articles every year. These codes are usually based on density functional theory (DFT). This modeling method uses multiple approximations to reduce the otherwise overwhelming complexity of calculating electron behavior according to the laws of quantum mechanics. The differences between the results obtained using the various codes result from the numerical approximations used and the numerical parameters on which they are based. These parameters are often tailored to the study of specific classes of materials or to the calculation of properties that are important for certain applications, for example conductivity for potential battery materials.
In their article recently published in the journal Nature Reviews Physics, the authors present the most comprehensive verification of DFT codes for solids to date. They provide the research community with tools and a set of guidelines for the evaluation and improvement of existing and future codes. The project was led and coordinated by the National Center for Computational Design and Discovery of Novel Materials (MARVEL) at the Swiss Federal Institute of Technology in Lausanne (EPFL, Switzerland). Several key contributions came from Prof. Thomas D. Kühne, founding director of the Center for Advanced Systems Understanding (CASUS) at the Helmholtz-Zentrum Dresden-Rossendorf (HZDR) and its science manager Dr. Hossein Mirhosseini. Both contributed their expertise as members of the CP2K development team. CP2K is an open-source software package that can perform atomistic simulations of solid, liquid, molecular, material, crystal and biological systems.
More elements, more crystal structures
The published work is a follow-up to a Science article from 2016, in which 40 different calculation approaches were compared with each other. For each approach, the energies of 71 crystals were calculated, each of which corresponded to an element of the periodic table. The study concluded that the most commonly used codes gave comparable results. “While this work was encouraging, it did not sufficiently account for chemical diversity,” says Dr. Giovanni Pizzi, head of the Materials Software and Data Group at the Paul Scherrer Institute PSI in Villigen (Switzerland) and lead author of the new publication. “In this study, we looked at 96 elements and simulated ten possible crystal structures for each of them.” Four different unaries (modifications) and six different oxides were examined for each of the first 96 elements in the periodic table. Unaries are crystals that only consist of atoms of one element. Oxides, on the other hand, also contain oxygen atoms. The result is a data set of 960 materials and their properties calculated with two independent all-electron DFT codes, FLEUR and WIEN2k, which explicitly consider all electrons of the atoms under consideration.
This data set can now be used by anyone as a reference to check the accuracy of other codes. This applies in particular to codes based on pseudopotentials. Here – in contrast to full electron codes – the electrons that are not involved in chemical bonds are treated in a simplified way, which makes the calculation easier. The authors compared the results of nine such pseudopotential codes with those from the full electron code dataset. “Together with other members of the CP2K team, we at CASUS were responsible for developing workflows and standard calculation protocols, as well as for performing calculations using two pseudopotential methods implemented in the CP2K software, namely Quickstep and SIRIUS,” says Mirhosseini. “This means that we compared the equations of state of all 960 crystals calculated with the two methods with the all-electron reference results. Our results could provide the basis for improving the methods implemented in CP2K,” he adds.
The study also contains a number of recommendations for those using DFT code. These include tips on how to ensure that calculation studies are reproducible, how to use the reference dataset to plan and perform verification studies, and how to extend the dataset to include other code families or material properties. “We hope that our dataset will serve as a reference for the research field for years to come,” says Pizzi.
Expanding the dataset, training the next generation
The results underline the importance of the reference dataset. The next goal is to expand it to include more structures and more complex properties that are calculated using more advanced functionals. And there is another challenge: instead of focusing solely on the precision of the different codes, the team plans to soon also consider the cost in terms of time and computing power. This would help others in the scientific community to find the most economical approach for their calculations.
Parallel to the technical development of code verification, a large consortium has already been formed that is not primarily concerned with molecules or codes. Rather, it has its sights set on the next generation: “We need to make PhD students and young scientists aware of the importance of validating and evaluating DFT modeling results,” says Kühne. “Therefore, the new consortium will develop the skills of these young people so that they are able to implement code verification processes in their research projects in the future. The benefits are obvious and include improved accuracy in general, as well as computational costs optimized to the desired precision.”
Adapted from a MARVEL press release
Publication
E. Bosoni, L. Beal, M. Bercx et al, How to verify the precision of density-functional-theory implementations via reproducible and universal workflows, Nature Reviews Physics, 2024 (DOI: 10.1038/s42254-023-00655-3)
Contact
Dr. Hossein Mirhosseini | Scientific Manager
Center for Advanced Systems Understanding (CASUS) at HZDR
Email: h.mirhosseini@hzdr.de
– – – – – –
Further links
👉 www.hzdr.de
Photo: Giovanni Pizzi, ETHL